Telomeres are the ends of eukaryotic chromosomes. They are composed of GT-rich repeated sequences, which are transcribed into non-coding RNAs and recruit specific proteins to protect DNA ends from fusions and degradations. This occurs mainly by preventing the DNA damage response (DDR) pathway from fully operating at telomeres. Telomeric sequences are lost at each passage of the replication fork and re-elongated by telomerase. In absence of this enzyme, telomeres shorten progressively at each cell division leading to a cell cycle arrest, which depends on the activation of the DDR at the shortest telomere(s). In many vertebrates, including humans, most somatic tissues do not express telomerase, telomeres shorten gradually, causing replicative senescence. In contrast, in mice, telomerase is active, and senescence has other causes. Still, the telomere shortening-dependent senescence phenotype can be recapitulated in mice or model unicellular eukaryotes in which telomerase is experimentally removed. Overall telomeres provide a unique mechanism to limit the lifespan of eukaryotic cells. In cancers, this pathway is eluded to allow limitless proliferation: the signaling of senescence is bypassed and, subsequently, telomere reduction is counteracted by abnormal re-elongation of telomeres. However, the mechanisms underlying these transitions are largely unknown.
An inherent difficulty in studying telomere biology is the heterogeneity resulting from intracellular differences in the length and status of telomeres and the immense intercellular variations in telomere-related phenotypes such as replicative senescence. Moreover, averaging data from heterogeneous cell populations results in biased models that often stand as enduring dogmas. Conversely, differences in telomere lengths and status might well be the source of cell-to-cell variations in replicative senescence onset, but this has not been extensively investigated. We thus propose to dissect the structure of telomeres at the single-molecule level and to connect this to the capacity of single cells to divide.
In this regard, we recently developed a microfluidics system to track single-cell lineages of Saccharomyces cerevisiae. In these otherwise immortal cells, removal of telomerase induces senescence and thus allows us to track senescence kinetics of single-cell lineages from onset until death. Our analysis has overturned the long held belief that replicative senescence is caused by a progressive increase in cell-cycle duration. While some cells undergo an abrupt switch from proliferative state to arrest, we also uncovered an unanticipated novel phenotype of telomerase deletion that reveals a new layer of telomere control over the DNA damage checkpoints. In these lineages, cell cycle dynamics follows an erratic pattern due to stochastic telomeric damage. Notably, the new phenotype explains the eventual emergence with time of genomic instability telomerase-negative cell populations. These results provide a testable model to probe new telomere functions that affect cell fate decisions, and reinforce our view that studying telomere biology at the single-telomere and single-cell levels with high temporal resolution will undoubtedly foster new discoveries.
In this context, we wish to determine the basic mechanisms of telomere replication and repair at work to control telomere shortening rate and understand the cell-to-cell variations of cellular responses at the onset of replicative senescence.
Our specific goals are thus (1) to determine the complete choreography of the DNA end replication problem in normal or altered telomeres; (2) to relate specific telomere structures with cellular checkpoint activation states; and (3) to investigate the cellular response to telomerase inactivation in a more global manner by testing new connections to telomeres and exploring new fields.
Our multidisciplinary projects integrate novel concepts and state-of-the-art methodologies in biology, biophysics, and mathematics. In this perspective, we set up original and sophisticated cellular genetic systems using the budding yeast Saccharomyces cerevisiae as a model. In parallel, we maintain a constant and fruitful dialogue with biophysicists and mathematicians at several levels. In the long-term we wish to establish a molecular framework for understanding the consequences of telomere and senescence variability not only for the evolution of molecular genetics of eukaryotes and the emergence of multi-cellularity but also for cellular aging and carcinogenesis.
Funding
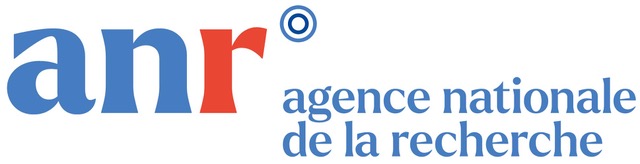
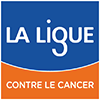
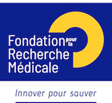
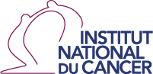